
Microscopic view of the Epstein-Barr virus (EBV), stained with Hematoxylin and eosin (HE). This color image highlights abnormal cells or tissue associated with EBV, a virus linked to several types of cancer and other disorders. Source: National Cancer Institute.
Recent research has indicated a link between Epstein-Barr virus (EBV) and multiple sclerosis (MS), with some researchers going as far as to say that EBV might be a potential trigger of MS. However, it remains unclear whether the virus also drives the progression of the disease. Current treatments focus largely on moderating inflammation. In this article, we expand on the hypothesized correlation between MS and EBV, and underscore the need to consider antiviral protection in drug development.
Background
Multiple sclerosis. MS is an unpredictable and debilitating neurological autoimmune disease that turns the body’s immune system against itself, explicitly targeting the central nervous system (CNS), including the brain, spinal cord and optic nerve. It is a complex patchwork of symptoms, outcomes, and experiences with unknown etiology and subject to personal resilience. The immune system mistakenly attacks the protective myelin that coats nerve fibers, causing communication disruptions between the brain and the rest of the body. While the cause of MS is still not fully understood, it is believed to involve a complex interplay of genetic and environmental factors, as well as medical history.
Symptoms of MS vary widely and depend on the amount of nerve damage and which nerves are affected. MS can be a silent companion for years, flaring up sporadically with bouts of symptoms known as relapsing MS. On the other end, there are also progressive forms characterized by steadily worsening in neurologic function. Yet, the progression and severity of MS vary broadly, making it an unpredictable disease.
Epstein-Barr virus
EBV is a common and widespread human virus from the herpesvirus family: approximately 9.5 out of 10 adults are infected with EBV (Kuri et al., 2020). It infects and establishes a lifelong persistence in the human body, primarily targeting B lymphocytes, a type of white blood cell. EBV is primarily transmitted through saliva and can spread through other means, such as blood transfusions and organ transplants.
Once the virus enters the body, it initially replicates within the throat and mouth epithelial cells (Hutt-Fletcher, 2014). This early infection often goes unnoticed or results in mild flu-like symptoms, making it challenging to diagnose. In most cases, the immune system successfully controls EBV, and the virus genome remains stationary within B lymphocytes. However, certain factors, such as a weakened immune system or genetic predisposition, can reactivate the virus at irregular intervals (see figure 1), leading to various complications (Kerr, 2019), ranging from mild to severe, depending on the individual’s immune response and overall health.
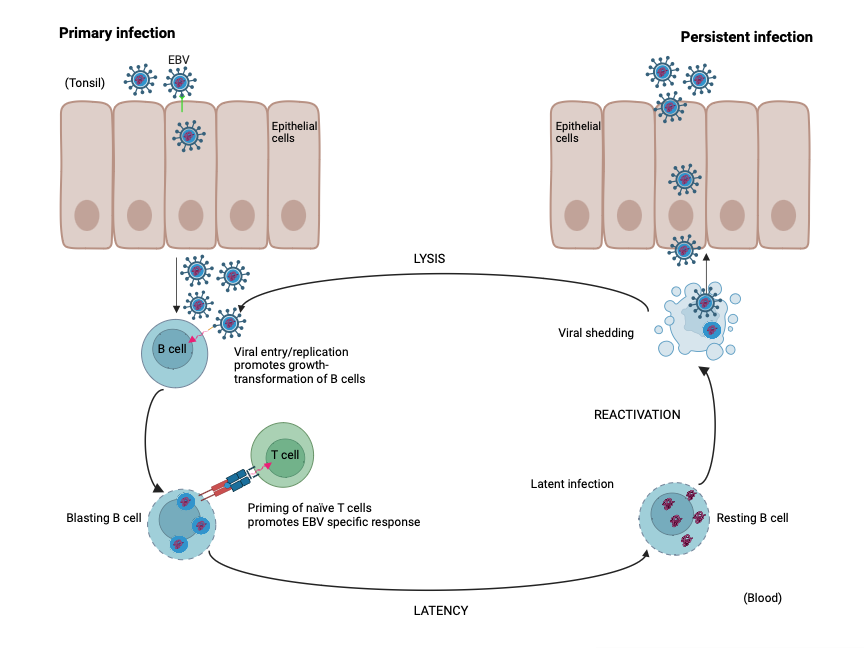
Figure 1: Replication cycle of EBV. Adapted from Odumade et al. (2011)
Connection Between EBV and MS
U.S. study found a strong link between MS and EBV. In 2022, a large US study provided evidence that suggests EBV infection is a crucial factor in the development of MS (Bjornevik et al., 2022). The study drew upon vast data from over 10 million US military personnel who served between 1993 and 2013. By examining the medical records of these individuals, the study determined who had EBV infections during childhood and as adults, and when MS symptoms first appeared. Additionally, since regular blood samples were collected from US soldiers, the study was able to identify the presence of an MS-related biomarker at specific times.
During the observation period, nearly 1,000 soldiers were identified as having developed MS. Out of these cases, 801 had available blood samples for analysis. In 800 out of 801 cases, antibodies against EBV were detected before the onset of MS symptoms. The presence of an MS biomarker was also found in military members who later developed the disease and has been discussed in that study as a potential marker for pre-symptomatic disease onset.
The majority of soldiers were already infected with EBV when they joined the army. However, 35 individuals with MS initially showed no evidence of the virus. Among them, 34 individuals were first infected with EBV as adults during their military service. Notably, not everyone who was EBV-positive developed MS. Nevertheless, statistical analyses revealed that EBV infection as an adult was associated with a 32-fold increased risk of MS. Other viral infections did not appear to impact the likelihood of developing MS. (See figure 2)
While these findings are based on observations and are not definitively conclusive, the large number of participants and the compelling statistical results strongly support the notion that EBV is a necessary condition and trigger for the development of MS.
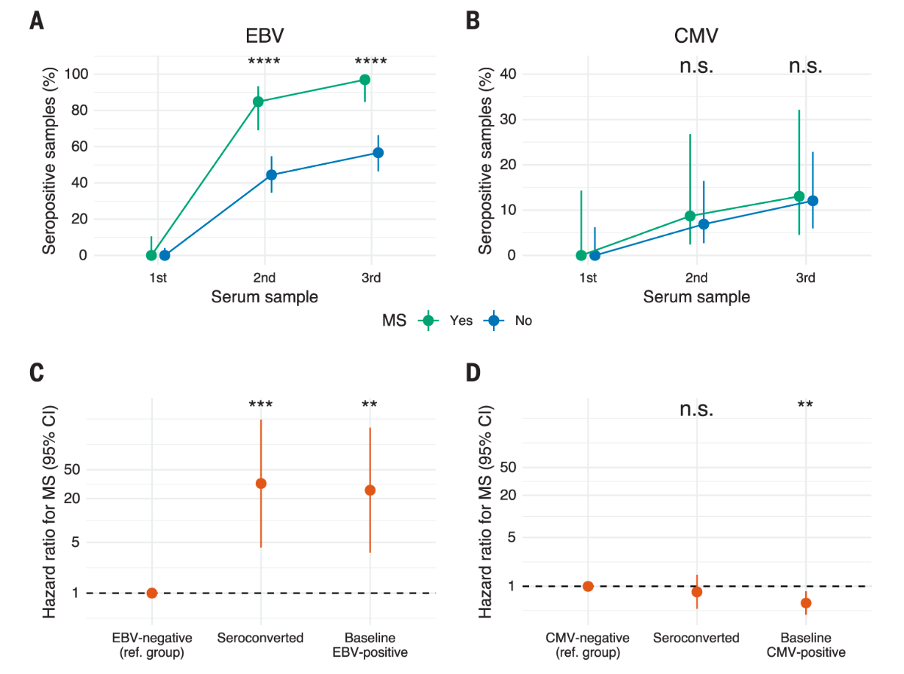
Figure 2: Study results depicting EBV as a trigger for MS onset. Bjornevik et al. (2022)
The Role of EBV in MS
EBV has been implicated in its impact on MS. The virus preferentially infects B lymphocytes. Infection alters the behavior of B lymphocytes and promotes inflammatory responses that could damage the nervous system. In addition, EBV overrides natural control mechanisms, potentially increasing the survival of B lymphocytes including the ones that could attack the nerve tissue. By doing so, EBV may prompt an immune response which in turn may damage the brain.
A further link between EBV and MS susceptibility was suggested by De Jager et al. (2008). In a genome-wide association study, MS patients carrying a genetic variant in HLA-DRB1, a gene involved in the recognition of proteins from invaders versus our body’s own proteins, and having high antibody levels against an EBV-related protein showed an increased risk of developing MS.
Another study (Lanz et al., 2022) suggests the presence of EBV-directed immune cells in the brains of MS patients and, therefore, an ongoing immune response against EBV. Active immune responses in the brain are considered risky because they can affect the surrounding nerve tissue.
Is EBV a Catalyst for MS?
A crucial question is whether EBV plays a role as a catalyst for MS. Does the existence of EBV and its potential periodic reactivation exacerbate the disease once it has been initiated? This hypothesis holds merit due to the persistent nature of EBV infection, wherein the virus persists in the body throughout one’s lifetime and sporadically reactivates, prompting recurrent immune reactions.
Indeed, researchers have uncovered evidence of an ongoing immune response against EBV among MS patients (Schneider-Hohendorf et al., 2022). Specific immune cells responsible for fighting viruses appear to monitor and react to the presence of active EBV infection continuously. This immune response against EBV reactivation may contribute to the deterioration of nerve tissue. In contrast, these same cells in the control groups did not exhibit the same extent of immune cell activity/reactivity, assuming absence of EBV reactivation.
A hallmark of MS is a distinctive pattern of antibodies in the cerebrospinal fluid called oligoclonal bands (OCBs). Within these bands, antibodies directed against EBV, nerve proteins or both (cross-reactivity) can be found. A recent study (Lanz et al., 2022) showed that, during maturation of antibodies directed to EBV, cross-reactivity can occur. Thus, an immune response against EBV can eventually turn against the patient’s body, potentially sparking disease progression and ongoing neurodegenerative processes.
Treatment of MS
Current Treatment Options for MS. MS is categorized in four main disease forms: Clinically Isolated Syndrome (CIS), relapsing-remitting MS (RRMS), primary progressive MS (PPMS) and secondary progressive MS (SPMS), each of which corresponds to a different level of severity and progression. RRMS is the most common form, with approximately 85% of MS patients expected developing RRMS (Klineova/Lublin 2018).
Currently, there are two main approaches to treating RRMS: Firstly, short-term glucocorticoids are used to relieve the acute relapses that characterize the early phases of the disease. Secondly, there are long-term disease-modifying therapies (DMT), which have varying mechanisms of action, but generally target the underlying immune system dysfunction associated with the disease to prevent relapses in the first place.
The initial treatments for MS patients are often beta interferons, including interferon beta-1a and -1b, or glatiramer acetate, which are given by injection and reduce inflammation in the CNS by suppressing the function of specific immune cells. For patients requiring more advanced treatment options, there are several options approved for MS, including:
- General inhibition of inflammatory reactions: dimethyl fumarate, cladribine (both oral), alemtuzumab (intravenous)
- Targeted elimination of activated lymphocytes: teriflunomide (oral)
- Preventing immune cells from entering the brain: natalizumab (intravenous), sphingosine-1 phosphate (S1P) receptor modulators fingolimod, ponesimod, siponimod, ozanimod (all oral)
- Elimination of B lymphocytes: rituximab, ocrelizumab, ublituximab (all intravenous), ofatumumab (injection)
Current therapies for RRMS primarily attempt to suppress relapses — they target the immune system to slow down its attacks on the body’s own nervous system. However, a recent study, comprising a meta-analysis of clinical data from over 35,000 MS patients, pointed towards the existence of a “smoldering disease” that leads to progression independent of relapse activity (PIRA) (Lublin et al., 2022). PIRA is seen as the primary factor in the progression of MS. The study also discovered that nearly half of the disability accumulation in RRMS patients was not associated with relapse activity, implying that PIRA contributes to half of the disease progression’s disability in RRMS.
Additionally, the study observed that, while many current drugs could delay or prevent disability related to relapse activity, they were unsuccessful in mitigating PIRA’s effects. Therefore, the halt of disability progression unrelated to relapse is still an unmet need for the MS patient population. This is reflected in the fact that only one treatment, ocrelizumab, is approved for PPMS, and even no treatments are approved for non-active SPMS.
Preventing relapses is not enough: New treatment options
A range of drugs has been approved for the treatment of MS; however, none of them specifically target the causes of MS, but rather inhibit the immune system in general. This may be one reason why the effectiveness of these therapies remains limited. It may also explain the frequent side effects that cause many people to refuse long-term treatment. In addition, the drugs are designed primarily to prevent relapses. This is a great relief for people with MS, but they can only halt the progression of the disease to a limited extent.
Interventions currently under investigation include:
- Bruton’s tyrosine kinase (BTK) inhibitors: BTK inhibitors such as tolebrutinib, evobrutinib, orelabrutinib or fenebrutinib are orally administered and aim to stop B cells and microglia from attacking myelin by modulating these cells.
- Mesenchymal Stem Cells (MSCs): While a precise mechanism of action in MS is yet to be clinically established, MSC is known to have immunomodulatory and regenerative properties. These therapies are thought to reset MS patients’ immune system by removing own stem cells and reconstituting it with healthy stem cells.
- Addressing multiple drivers of neurodegeneration: Vidofludimus calcium is a selective immune modulator offering a dual mechanism via nuclear receptor-related 1 (Nurr1) modulation and dihydroorotate dehydrogenase (DHODH) inhibition. The activation of the transcription factor Nurr1 is related to direct neuroprotective properties. The DHODH pathway, a key enzyme in the metabolism of overactive immune cells and virus-infected cells, is associated with anti-inflammatory and anti-viral effects.
If the assumption that EBV is a driver of MS is correct, in theory, a highly effective vaccine could largely prevent the development of MS. However, it is considered unlikely that a vaccine could completely prevent exposure to EBV, but other approaches are conceivable. These include agents that prevent reactivation of EBV or at least suppress the immune response to EBV. For better understanding, clinical trials should investigate the impact of their agent on EBV.
Conclusion: Validating the role of Epstein-Barr virus in multiple sclerosis
This article highlighted the recently revealed role of EBV in the etiopathogenesis of MS, and although the clear causality has not yet been established, these findings change the understanding of the disease and introduce a new variable into the equation. The new evidence validates the link between MS and EBV infection, providing a new perspective on therapeutic strategies to mitigate the burden of the disease.
Despite these advancements in understanding the drivers for MS, current therapies, although efficacious in managing disease relapses, fall short in addressing the critical unmet medical need: the progression of disability in MS patients. DMTs have changed the clinical landscape of MS, but their efficacy remains primarily focused on the inflammatory aspect of the disease, offering limited assistance to neurodegenerative aspects. Hence, it is crucial to focus efforts on discovering and developing novel therapeutic strategies that aim at both limiting inflammation and halt or slow the disability progression in MS patients by promoting neuroprotection and repair.
Author bios
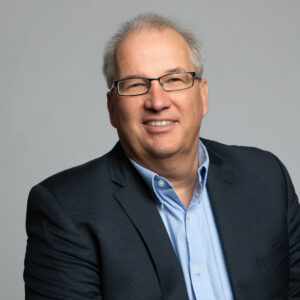
Dr. Andreas Muehler
Andreas Muehler, M.D., M.B.A., joined Immunic in August 2016 as Chief Medical Officer. From 2009 until joining Immunic, Dr. Muehler was an independent medical consultant, serving in key interim leadership roles at a number of pharmaceutical and medical device companies. Earlier in his career, he served at the helm of several U.S. based companies including President and Chief Executive Officer of MicroMRI, Inc., President of CAD Sciences, Chief Executive Officer and Vice President, Corporate Development at Cellectar Inc. as well several roles spanning preclinical and clinical development, business development, as well as licensing and marketing at Berlex Laboratories. Before joining the industry, Dr. Muehler focused on academic research at the University of California, San Francisco. Dr. Muehler received an M.D. from Humboldt-University (Charité) in Berlin, Germany, and an M.B.A. from Duke University in Durham, North Carolina.
Hella Kohlhof, Ph.D., joined Immunic in January 2017 as Chief Scientific Officer. In 2008, Dr. Kohlhof joined 4SC AG and held several positions, including Director of Development Projects, where she was responsible for the entire product development pipeline of 4SC. Dr. Kohlhof studied biology in Aachen and Munich, Germany, and Gothenburg, Sweden. She received her doctorate in biology from the Ludwig Maximilians University of Munich and the Institute of Clinical Molecular Biology and Tumor Genetics at the Helmholtz Centre in Munich.
Evelyn Peelen, Ph.D, joined Immunic Therapeutics in 2019. As Senior Manager Translational Pharmacology she is overseeing the primary pharmacology studies. Before, she has worked in academia, first as a PhD student at the University of Maastricht, The Netherlands, then as a postdoctoral fellow at the Biomedical Research Institute, Hasselt University, Belgium, and lastly as a postdoctoral fellow at the Research Center of the Centre Hospitalier de L’Université de Montréal (CHUM), Canada. Her research in academia was focused on the immune system in MS. Dr. Peelen graduated in molecular life sciences from the Radboud University Nijmegen, The Netherlands.
References
- Bjornevik, K., Cortese, M., Healy, B. C., Kuhle, J., Mina, M. J., Leng, Y., Elledge, S. J., Niebuhr, D. W., Scher, A. I., Munger, K. L., & Ascherio, A. (2022). Longitudinal analysis reveals high prevalence of Epstein-Barr virus associated with multiple sclerosis. Science, 375(6578), 296–301. https://doi.org/10.1126/science.abj8222
- De Jager, P. L., Simon, K., Münger, K., Rioux, J. D., Hafler, D. A., & Ascherio, A. (2008). Integrating risk factors: HLA-DRB1*1501 and Epstein-Barr virus in multiple sclerosis. Neurology, 70(Issue 13, Part 2), 1113–1118. https://doi.org/10.1212/01.wnl.0000294325.63006.f8
- Hutt-Fletcher, L. M. (2014). Epstein–Barr virus replicating in epithelial cells. Proceedings of the National Academy of Sciences of the United States of America, 111(46), 16242–16243. https://doi.org/10.1073/pnas.1418974111
- Kerr, J. R. (2019). Epstein-Barr virus (EBV) reactivation and therapeutic inhibitors. Journal of Clinical Pathology, 72(10), 651–658. https://doi.org/10.1136/jclinpath-2019-205822
- Klineova, S., & Lublin, F. D. (2018). Clinical Course of Multiple Sclerosis. Cold Spring Harbor perspectives in medicine, 8(9), a028928. https://doi.org/10.1101/cshperspect.a028928Kuri, A., Jacobs, B. M., Vickaryous, N., Pakpoor, J., Middeldorp, J., Giovannoni, G., & Dobson, R. (2020). Epidemiology of Epstein-Barr virus infection and infectious mononucleosis in the United Kingdom. BMC public health, 20(1), 912. https://doi.org/10.1186/s12889-020-09049-x
- Lanz, T. V., Brewer, R. C., Ho, P. P., Moon, J., Jude, K., Fernandez, D., Fernandes, R. J., Gomez, A. M., Nadj, G., Bartley, C. M., Schubert, R., Hawes, I. A., Vazquez, S. R., Iyer, M., Zuchero, J. B., Teegen, B., Dunn, J., Lock, C., Kipp, L., . . . Robinson, W. H. (2022). Clonally expanded B cells in multiple sclerosis bind EBV EBNA1 and GlialCAM. Nature, 603(7900), 321–327. https://doi.org/10.1038/s41586-022-04432-7
- Lublin, F. D., Häring, D. A., Ganjgahi, H., Ocampo, A., Hatami, F., Čuklina, J., Aarden, P., Dahlke, F., Arnold, D. L., Wiendl, H., Chitnis, T., Nichols, T. E., Kieseier, B. C., & Bermel, R. A. (2022). How patients with multiple sclerosis acquire disability. Brain, 145(9), 3147–3161. https://doi.org/10.1093/brain/awac016
- Odumade, O. A., Hogquist, K. A., & Balfour, H. H. (2011). Progress and problems in understanding and managing primary Epstein-Barr virus infections. Clinical Microbiology Reviews, 24(1), 193–209. https://doi.org/10.1128/cmr.00044-10
- Schneider-Hohendorf, T., Gerdes, L. A., Pignolet, B., Gittelman, R. M., Ostkamp, P., Rubelt, F., Raposo, C., Tackenberg, B., Riepenhausen, M., Janoschka, C., Wünsch, C., Bucciarelli, F., Flierl-Hecht, A., Beltrán, E., Kümpfel, T., Anslinger, K., Gross, C. C., Chapman, H. L., Kaplan, I., . . . Schwab, N. (2022). Broader Epstein–Barr virus–specific T cell receptor repertoire in patients with multiple sclerosis. Journal of Experimental Medicine, 219(11). https://doi.org/10.1084/jem.20220650